Click here to register!
Introduction to Airborne Wind Energy
Concept
Airborne Wind Energy (AWE) is the conversion of wind energy into electricity using automatic tethered flying devices. There are three main concepts:
- The ground-generation (“ground-gen”) pumping concept converts the pulling power of the flying devices over a winch and a generator on the ground.
- The on-board generation concept (“fly-gen”) uses the rotors of the aircraft to generate the power in the air in combination with a conducting tether.
- The rotary concept uses a lifter kite to hold up a flexible structure of multiple aerofoils which rotate and transmit the movement to the generator on the ground.[1][2][3]
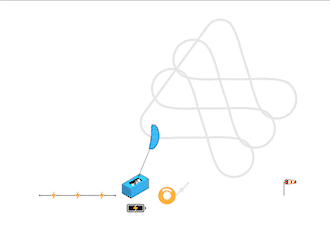
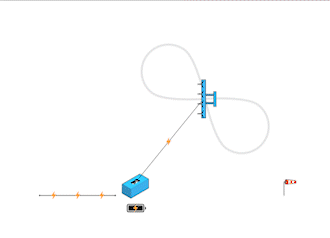
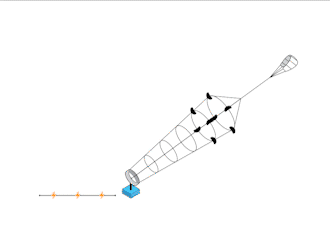
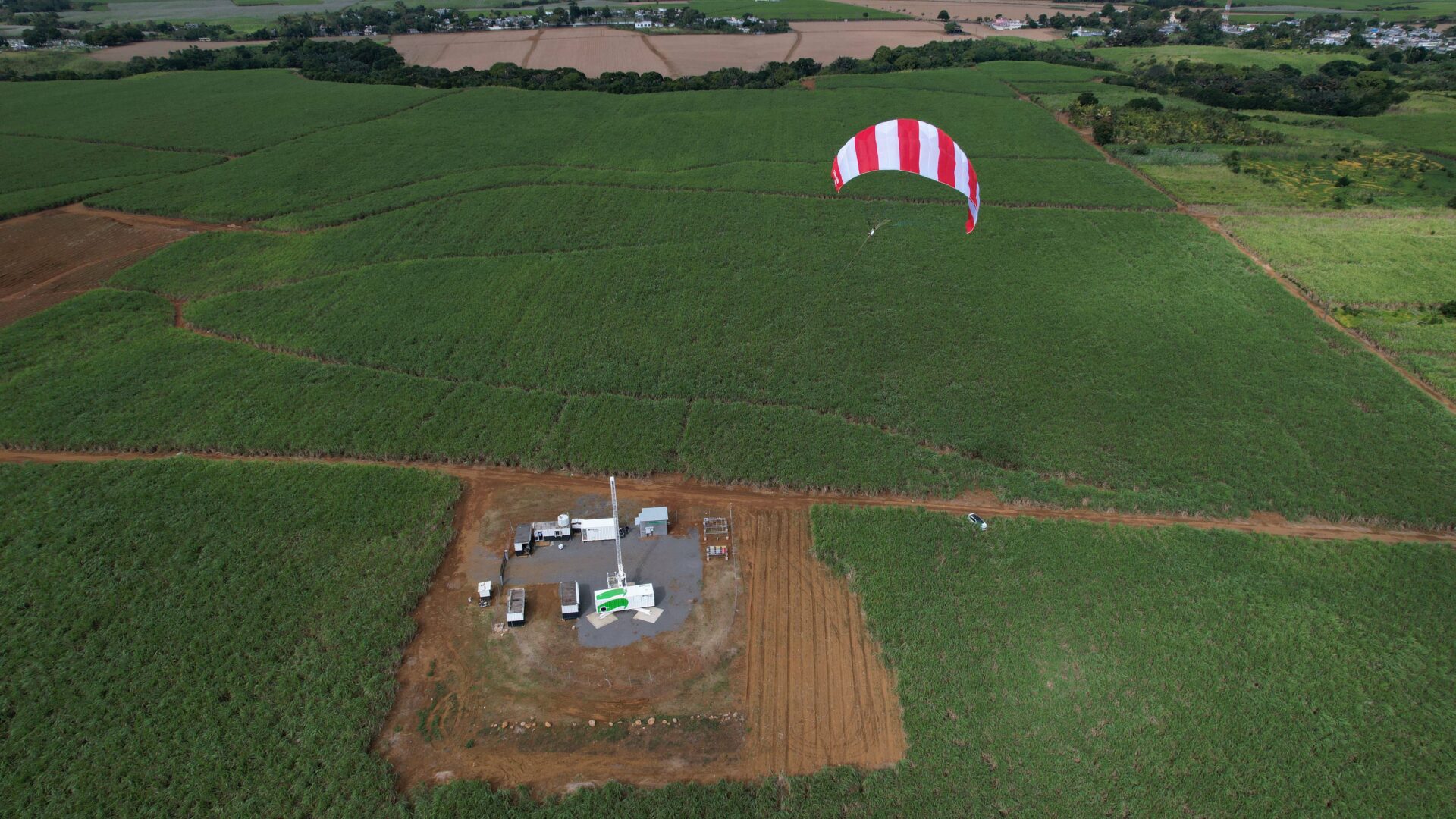
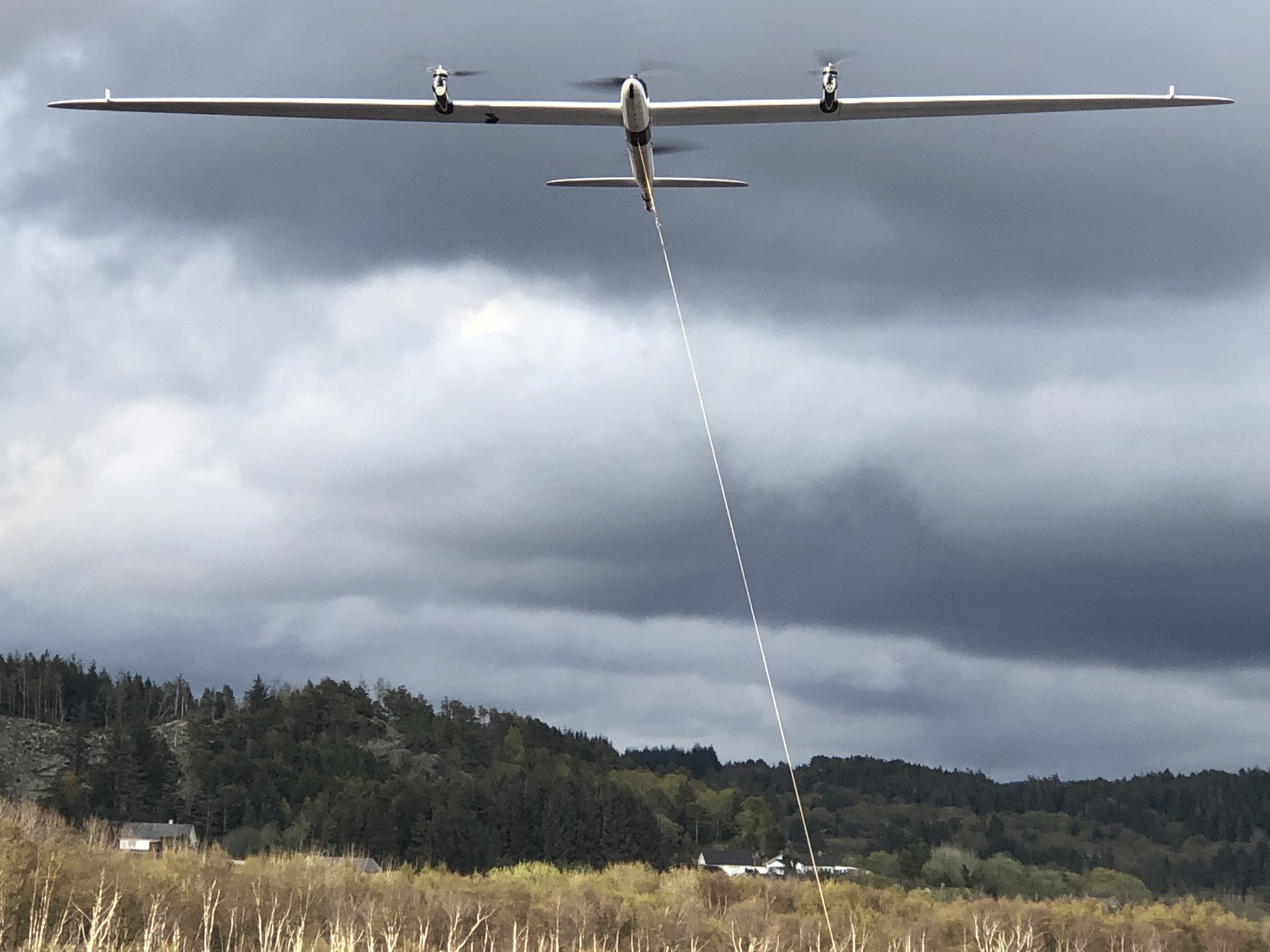
by Kitemill (Norway)
Advantages
There are a number of advantages of AWE systems:
- Low material use: Replacing the tower of a wind turbine by a lightweight tether substantially reduces the material consumption by up to 90%, thus decreasing the environmental impact with regards to the carbon footprint over the life-cycle[4] as well as reducing visual impacts.
- Additional wind resource: Wind at higher altitudes is an energy resource that has not been exploited so far. In 2018, the European Commission presented a sector study which acknowledges the potential of AWE to supply up to 100% of EU electricity demand with 1% of land use[5]. The maps show average wind speeds in Europe at typical hub height of wind turbines of 100m and at variable altitude of up to 500 m (right)[6]: Conditions that are usually only available offshore become accessible also onshore with AWE.
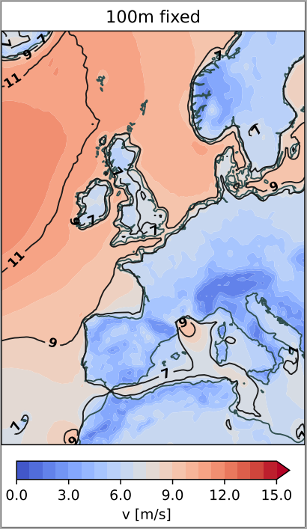
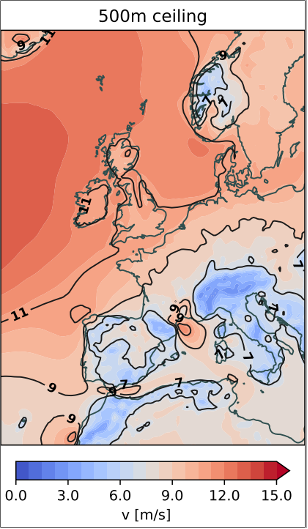
- High capacity factor: AWE allows for continuous adjustment of the harvesting altitude to the best available wind resource. This increases the complementarity to other renewables in both stand-alone but also hybrid installations with solar PV and thus provides benefits for energy system integration.
- Low costs: The decrease in capital costs (CAPEX) due to low material use, the increase in capacity factor, the easier logistics and quick set-up as well as the high power density per km2 can potentially lead to a substantial reduction of the levelized costs of wind energy (LCOE). The first commercial AWE systems are already today competitive in markets with diesel-based power generation.
- Access to new markets: Since AWE systems are scalable from a few kilowatt to several megawatt (several companies plan to upscale to 1-3 MW devices over the next years), many markets and locations can be accessed like offshore repowering, floating offshore, mountainous and remote locations.
Sector Development
Deemed a potentially game-changing solution[7][8], AWE is increasingly attracting the attention of governments, policy makers and industry worldwide. Over the last decade, AWE has developed from a pool of conceptual ideas and first small-scale experiments into a vibrant field of R&D, producing a diverse array of technology demonstrators and first commercial systems, ranging up to power outputs of 100-200 kW.
Today, there are about a dozen original equipment AWE technology developers, and world-wide more than 50 research institutes, universities, industrial associations, companies along the supply chain, and utilities are involved in the sector at different levels[9]. Every two years, the sector gathers at the AWE Conference series with over 200 delegates[10].
Critical technical challenges have been mastered, such as automatic energy harvesting, reliable sensors, and state estimation as well as developing tethered aircraft and kites for aerodynamic load cycles that are far more demanding than those for conventional aircraft and paragliders. Remaining challenges are the fully automated launching and landing, durable and lightweight materials to sustain a high number of load cycles, a systematically increased reliability and ensured operational safety including regulatory aspects.
The first companies have started commercialising their systems. Currently, they mainly target on remote and island off-grid markets. However, to successfully enter the highly competitive and regulated European electricity market, AWE will require specific policy support to reach its full potential like other renewable energy technologies in the past. Apart from R&D and investment support – which is to some extent already available and used at European and national level[11] – there will also be the need for revenue support schemes[12]. A roadmap of the AWE sector has been described in a White Paper by BVG Associates in 2022. It shows that about 1 GW could be deployed by the early 2030s and several hundred GW by 2050[13].
The sector association Airborne Wind Europe was founded in 2018 to bring together industry and academia, to represent the interests of the sector, and to work together on important topics like safety and technical guidelines, environmental impact etc. The IEA Wind Task 48 on Airborne Wind Energy was established in 2021[14]. This collaboration platform is supported by eleven countries (BE, CH, DE, DK, ES, IE, IT, NL, NO, UK, US) and several dozen organizations. Task 48 consists of five Work Packages: i) Resource potential and markets; ii) reference models, tools and metrics; iii) safety and regulation; iv) Social Acceptance; v) AWES Architectures. You can visit Airborne Wind Europe here.
References
- ↑ A. Cherubini, A. Papini, R. Vertechy, M. Fontana, Airborne Wind Energy Systems: A review of the technologies. Renewable and Sustainable Energy Reviews 51, 1461–476, 2015. doi:10.1016/j.rser.2015.07.053
- ↑ R. Schmehl, Ed., Airborne Wind Energy - Advances in Technology Development and Research. Singapore: Springer, 2018. doi:10.1007/978-981-10-1947-0
- ↑ V. Nelson, Innovative Wind Turbines: An Illustrated Guidebook,. CRC Press, 2019. ISBN 9780367819316
- ↑ S. Wilhelm, Life Cycle Assessment of Electricity Production from Airborne Wind Energy, https://link.springer.com/chapter/10.1007/978-981-10-1947-0_30, 2018
- ↑ K. van Hussen et al, Study on challenges in the commercialisation of airborne wind energy systems, prepared by Ecorys BV for the European Commission’s DG Research and Innovation, Sept 2018. doi:10.2777/87591
- ↑ Adapted from Bechtle et. Al , Airborne Wind Energy Resource Analysis, 2018, arXiv:1808.07718(physics.ao-ph)
- ↑ IRENA: Innovation Outlook: Off-shore Wind, International Renewable Energy Agency, Abu Dhabi, 2016.
- ↑ IRENA: Future of wind: Deployment, investment, technology, grid integration and socio-economic aspects, Abu Dhabi, 2019.
- ↑ R. Schmehl: AWE R&D landscape 2018. http://www.awesco.eu/awe-explained/#gallery-4 Accessed 6 April 2020
- ↑ See www.awec2015.com, www.awec2017.com, www.awec2019.com, www.awec2021.com, www.awec2024.com
- ↑ E.g. Horizon 2020 projects AWESCO (2015-18) or Interreg NWE MegaAWE (2020-23)
- ↑ K. Petrick, Policies for Airborne Wind Energy - Preparing the grounds for AWE-specific incentive schemes, 2018, https://airbornewindeurope.org/policies-for-airborne-wind-energy-scoping-study-now-in-the-eu-open-access-repository/
- ↑ BVGA-Getting-Airborne-White-Paper-220929.pdf (airbornewindeurope.org)
- ↑ www.iea-wind.org/task48